Spinal Cord Injury & Plasticity
It is estimated that there are more than 180,000 new cases of spinal cord injury that occur world-wide each year. Around 17,700 of those occur within the United States of America. In addition, it is estimated that there are between 250,000 and 360,000 people living with spinal cord injury in the US alone. The majority of SCI’s (estimated at ~59% of injuries in 2017) occur at ‘cervical’ levels, or the level of the neck. Injuries at this level result in the most devastating outcomes, including impaired use of upper and lower extremities (arms and legs), impaired breathing, bladder and bowel function, and increased risk of chronic pain to name a few.
The National Spinal Cord Injury Statistical Center offers information on spinal cord injury statistics
Plasticity, or more specifically in the present circumstance ‘neuroplasticity’, is a functional or anatomical change that can occur either spontaneously or be therapeutically driven, and will likely become permanent without some intervention. For example, some limited spontaneous functional improvements can occur following spinal cord injury. This spontaneous recovery – or functional plasticity – may be due to coinciding anatomical changes.
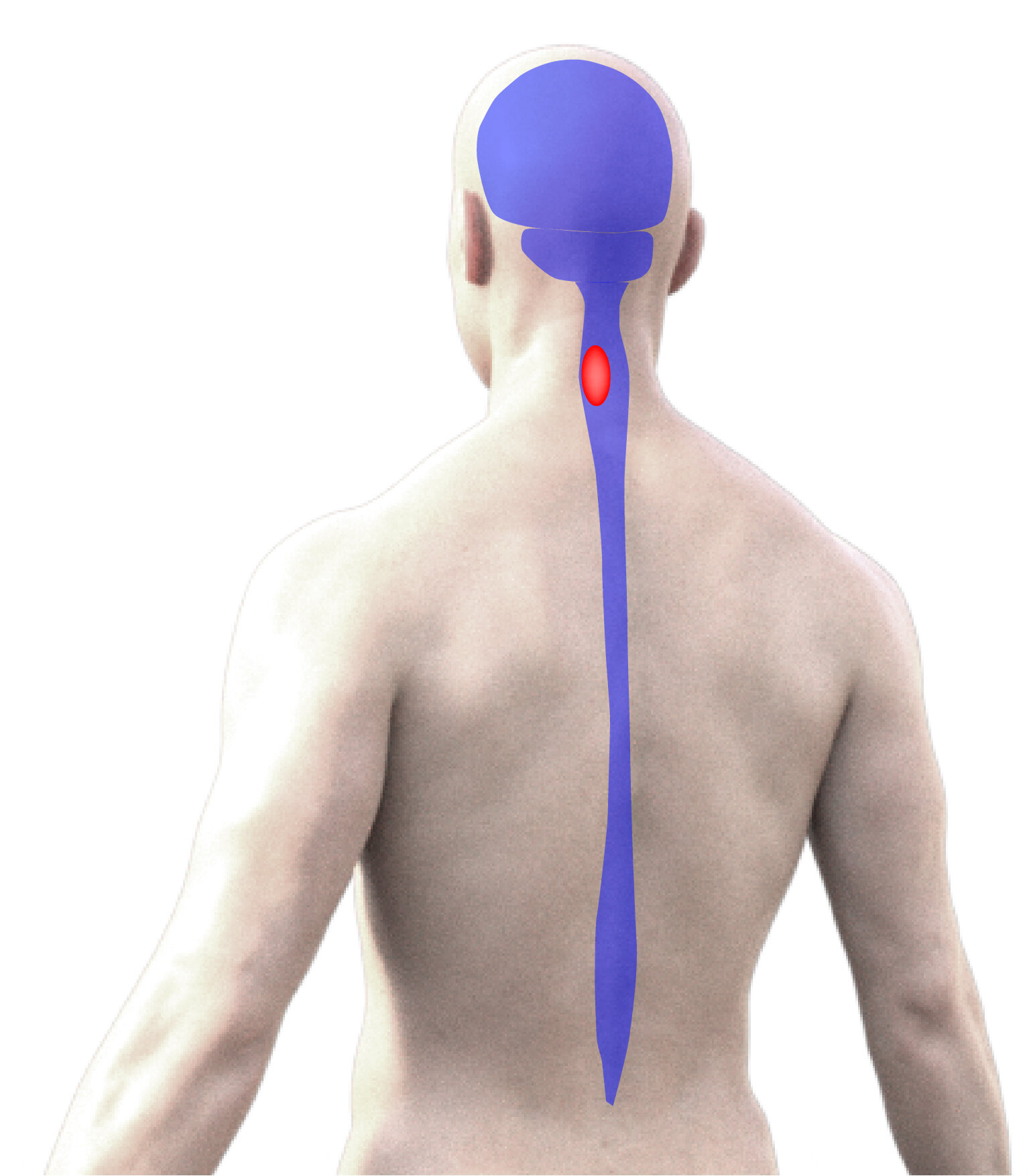
Our research team is investigating the effects of cervical spinal cord injury (SCI) and how recovery can be optimized. A primary focus of this work on the functional consequences of cervical SCI (in particular how breathing and upper extremity (arm) function is impaired) and what potential there is for progressive, spontaneous functional recovery – or functional ‘plasticity’. We are also developing and testing strategies for promoting beneficial plasticity and recovery following cervical SCI.
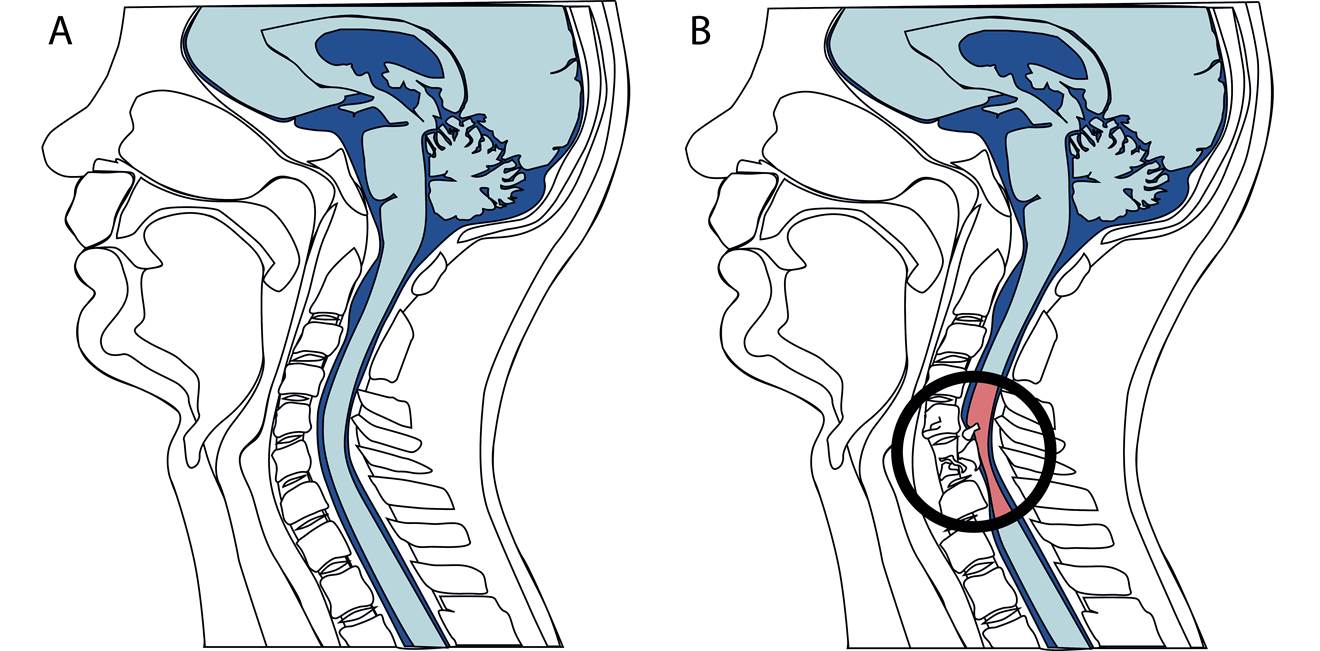
This schematic diagram is comparable to the images that are obtained with magnetic resonance imaging (MRI), showing a section through the human head and neck. Highlighted in blue are the brain (top, which sits behind the eyes and nose) and the and spinal cord (beneath the brain). While the image in A shows an intact brain and spinal cord, B is an illustration of what might happen following a spinal cord injury at the cervical level. Bone surrounding the spinal cord (vertebrae) can impact (or contuse) and compress the spinal cord. If the bone fractures, the bone fragments may penetrate the spinal cord tissue causing additional damage.
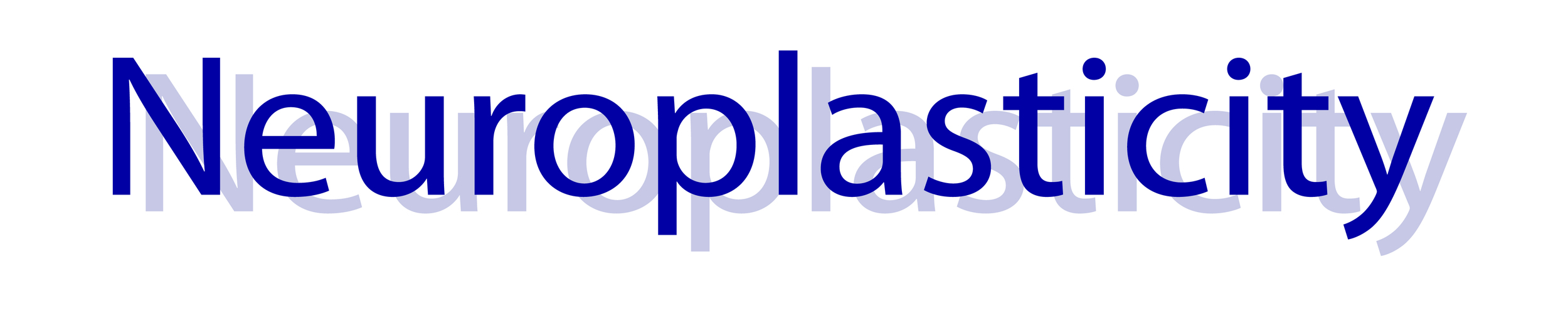
Using a range of neuroanatomical, neurophysiological and behavioural approaches, our research has helped to define the neural circuitry in the normal (uninjured) spinal cord, how this circuitry is affected by spinal cord injury and how treatments can be used to promote repair. A particular focus of our research has been on the role of spinal interneurons in mediating normal motor function, and spontaneous or therapeutically enhanced functional plasticity following spinal cord injury. Several studies have shown that spinal interneurons are involved with spontaneous reorganization of neuronal circuitry, which can provide new anatomical pathways capable of facilitating functional recovery. These interneurons – interspersed throughout spinal cord – can receive input from neurons in the brain and then themselves make contact with neurons below the spinal cord injury. Thus, they can relay information from the brain, around the injury, to neurons below the injury that control the muscles. While this remodeling of connections can occur spontaneously, it may also be enhanced by some developing therapeutic strategies (see also the recent TED talk by Gregoire Courtine). While there is a growing appreciation among scientists and clinicians that spinal interneurons may be essential to plasticity and recovery after spinal cord injury, little is known about their distribution or how they aid functional improvement.
While there a number of research laboratories investigating neuroplasticity after spinal cord injury, and the number continues to grow, little effort has been made to investigate changes that occur above the spinal cord in the brain and brainstem (supraspinal regions). Our research team is now investigating spontaneous functional and anatomical changes that may arise within supraspinal regions following a cervical spinal cord injury. While cortical plasticity following SCI is a rapidly growing area of research, much less is known about whether such potential also exists in brainstem regions. Using combined anatomical and functional techniques, we have begun characterizing functional reorganization in the medulla. In particular, I am examining alterations in the activity of respiratory neurons in the medulla following cervical SCI and assessing how such changes may affect the potential for recovery.
Breathing after Injury
One of the most devastating consequences of cervical spinal cord injury is impaired breathing. Respiratory deficits post-injury are a leading contributor to morbidity and mortality in injured individuals
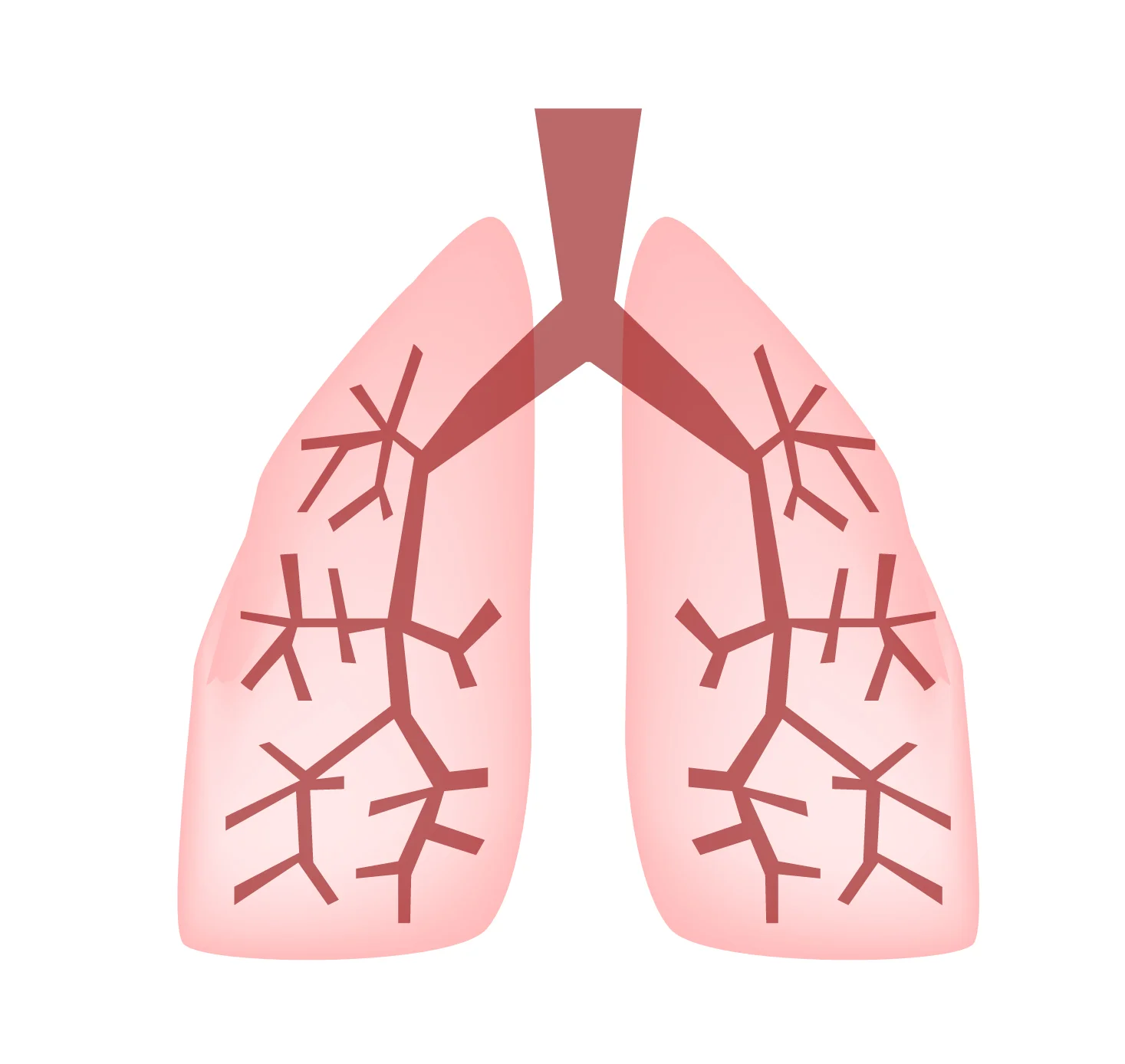
Maintenance of life among higher vertebrates depends on permanent, rhythmic and coordinated activity of respiratory muscles. The act of drawing air in and out of the lungs is one of the most basic, life-sustaining behaviors that we perform. More than half of the spinal cord injuries that occur in people affect their ability to breath (respiratory function). Motoneurons in the spinal cord that innervate respiratory muscles can be damaged and lost following spinal cord injury, which affects muscle function. In addition, axons from the brain and brainstem that send respiratory signals to these spinal motoneurons can be damaged which impairs motoneuron (and thus muscle) function.
Respiratory muscles are innervated by neurons that are distributed throughout nearly the entire spinal cord (from the neck - or cervical levels - to lower spinal cord - or lumbar levels). This is illustrated in the figure below (taken from Lane (2011), Resp. Physiol. Neurobiol.) Therefore, an injury at nearly any level of the spinal cord can affect control of breathing in some way. The most devastating consequences occur following cervical level injuries. Injuries at this level compromise pathways from the brain to neurons at lower spinal cord levels. In addition, cervical level injuries can directly compromise the 'phrenic' motor system. Phrenic motor neurons innervate the diaphragm - often considered the main muscle of breathing. High or mid-cervical SCIs lead to impaired diaphragm function (paresis or paralysis). People with injuries at these levels often lose voluntary control of breathing and require 'assisted' ventilation (e.g. by mechanical ventilation, or diaphragm pacing). Assisted ventilation represents the most effective means of care for people unable to control breathing on their own, but several lines of research are now investigating whether there are ways to improve on these strategies, or alternative approaches to promoting recovery of breathing.
Our research team is studying how breathing – and activity in respiratory muscles - is affected by cervical SCI, exploring the potential for spontaneous neuroplasticity, and trying to identify therapeutic strategies capable of promoting improvement in respiratory function.
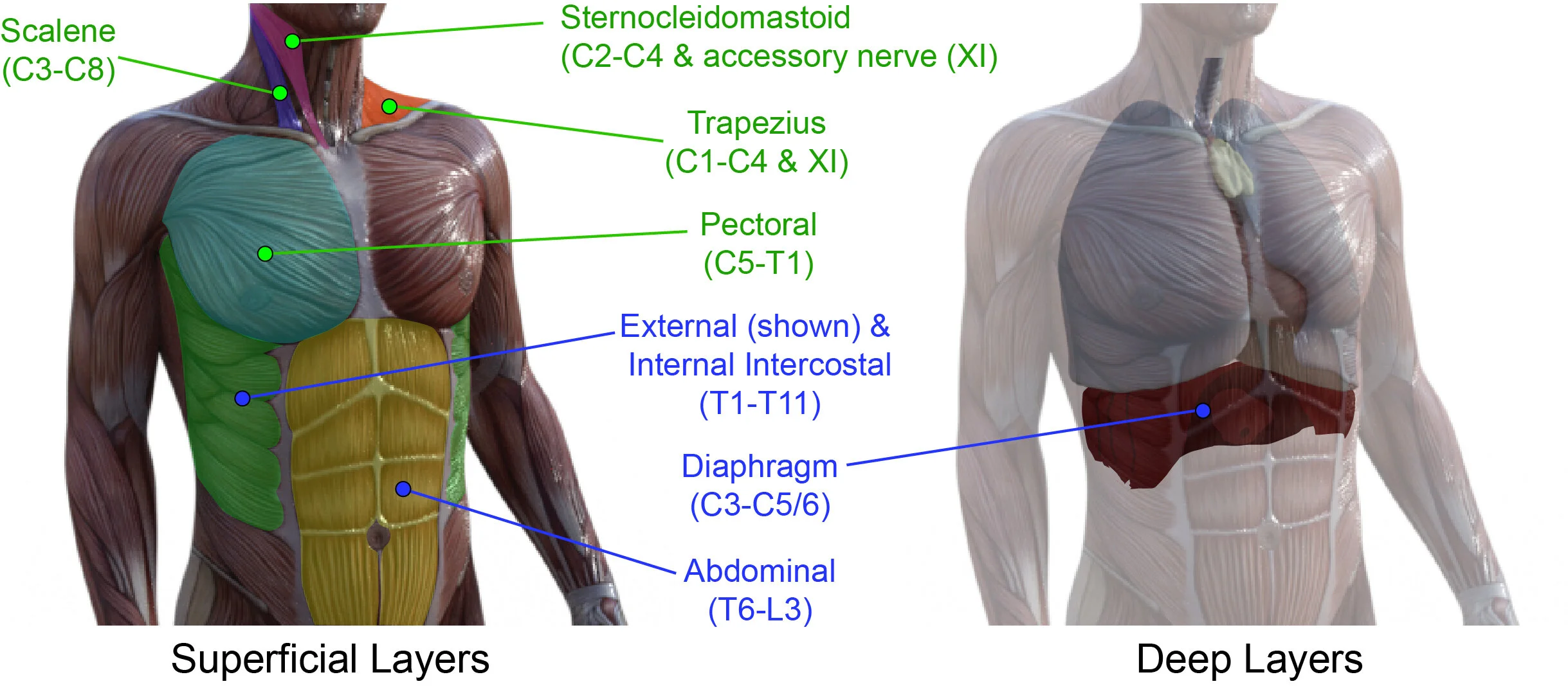
The schematic diagram above is taken from a published manuscript (Locke et al (2021) Neural Regeneration Research). The figure illustrates the muscles that can be recruited into breathing activity. Those highlighted as ‘primary respiratory muscles’ (in blue) are those that can active during normal breathing (at rest). This figure also indicates the spinal distribution of motor neurons that innervate each muscle, listed by the spinal region (cervical (C), thoracic (T) and lumbar (L)), and the spinal segment within those regions (#).

The above photo is taken from a published manuscript (Lane et al. (2008) Journal of Comparative Neurology). Our research team uses experimental models of cervical spinal cord injury to assess how breathing is impaired, explore the potential for spontaneous neuroplasticity, and examine how injuries disrupt the anatomical pathways that control respiratory function. Neuroanatomical tracing and histological methods are used to examine the distribution of respiratory neurons, the connections between them, and how these are affected by SCI. The photo above is one such example of these tracing studies. This image is taken from a histological section through the cervical spinal cord. The neurons in green are spinal motoneurons that innervate the diaphragm on one side of the body (hemi-diaphragm). The red fibers surrounding these cells are axons from the respiratory control neurons in the brainstem. These neurons in the brainstem send patterned activity to spinal motoneurons, which stimulate contraction of the diaphragm contributing to inhalation. Our team also uses electrophysiological methods to record the activity (or ‘firing’) of these neurons, the information sent along their axons, and the contractions of the respiratory muscles. This allows a very detailed assessment of how the respiratory pathways function following SCI. The coordinated contraction of inspiratory and expiratory muscles results in the patterned activity of breathing (inhalation and expiration) – or ventilation. Our research team uses plethysmography to assess ventilatory function following cervical SCI.
Developing Treatment Strategies
While it has long been thought that spinal cord injuries resulted in permanent paralysis, research has shown that a small amount of recovery – called plasticity - can occur naturally as the injured spinal cord attempts to rebuild itself. Although the extent of recovery is limited, treatments that strengthen this plasticity will likely lead to the effective therapeutic outcomes. The long-term goal of our research program is to harness and enhance neuroplasticity, and optimize lasting functional recovery.

Although promoting long-distance regrowth of injured axons has been a longstanding objective of spinal cord injury (SCI) research, there is now wide-spread appreciation for the contribution of intrinsic neuroplastic axonal growth (i.e., short-distance intraspinal sprouting) to spontaneous functional recovery. Spinal interneurons are identified as an essential component of this plasticity and contribute to the formation of new anatomical pathways following SCI, that can re-route information around the site of injury. In effect, such “by-pass” pathways constitute neural relays that contribute to functional improvements. Our research team believes that treatments capable of harnessing and enhancing this natural plasticity may circumvent the need for long-range axonal growth. Accordingly, we believe that spinal interneurons are a key therapeutic target for optimizing anatomical repair and functional recovery following SCI.
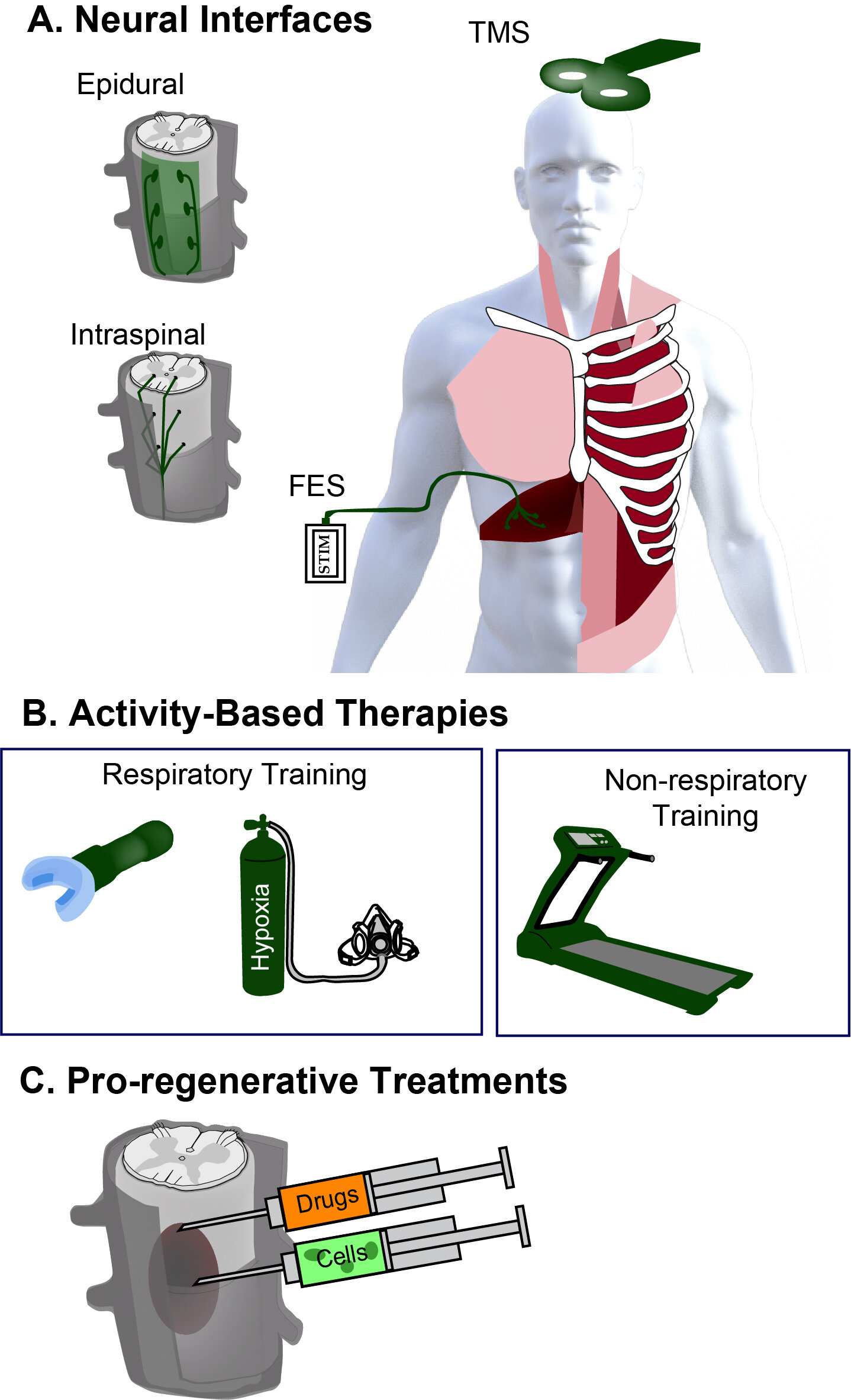
As the above figure highlights (taken from Locke et al. 2021), there are many different strategies being developed for treating the injured spinal cord, ranging from A. neural interfacing (brain-computer interfacing, neural/muscle stimulation), B. Activity-based therapies such as physical therapy and rehabilitation (treadmill training, respiratory training), and C. pro-regenerative strategies for enhancing tissue growth and repair 9drug delivery, gene therapy and cell transplantation). Our research program is exploring how treatments within these categories can promote plasticity and tissue repair to improve respiratory outcomes.
A key focus of several ongoing projects is on the use of ‘neural progenitor cell’ (the developing neuronal and glial building blocks of spinal cord) transplantation, which is capable of promoting spinal cord repair. This approach has been used extensively in the past 40 years in a wide range of SCI models (e.g. see works by Drs Victoria Moreno Manzano (CIPF, Spain), Paul Reier (Florida, USA), Itzhak Fischer (Drexel, USA), Mark Tuszynski (UCSD, USA), and Michael Fehlings, (UHN, Canada)), and other neurological disorders. Our research now builds upon this extensive experience to assess whether transplantation of neural progenitor cells - which are enriched with spinal interneurons - can repair respiratory pathways after cervical SCI and promote recovery of respiratory function. Our primary focus is on promoting repair of the injured cervical spinal cord.
In collaboration with Shane Liddelow (NYU), Shelly Sakiyama-Elbert (UTexas) and Lyandysha Zholudeva (Gladstone), our ongoing research is harnessing advanced cellular engineering methods to create refined populations of therapeutically relevant neurons and glia.
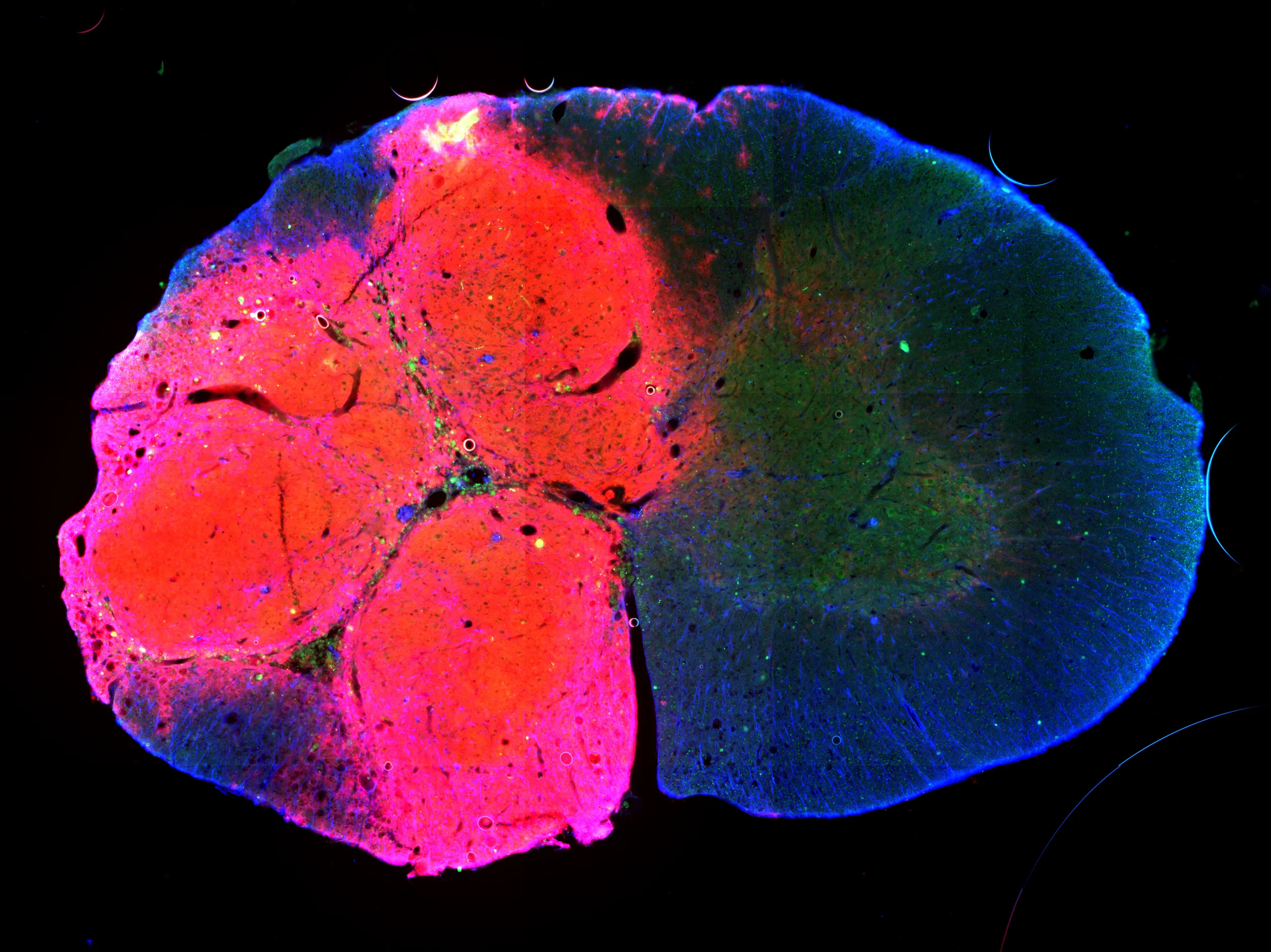
The above photo is a histological section taken through the cervical spinal cord (a cross-section) at the site of an injury (left side) that also received cell transplantation. The right half of this image shows spinal cord tissue that has been spared by this lateralized – or asymmetric – injury. The cells transplanted in these studies are labeled (in red here) so that we are able to track their distribution. This image confirms that the transplanted cells have survived and differentiated into neuronal and glial cells. These ongoing studies – now led by Tara Fortino and Adam Hall – are assessing whether transplantation of neuronal and glial precursor cells can restore continuity of the injured spinal cord, promote repair of phrenic pathways, and enhance recovery of diaphragm function after cervical SCI.